Sputnik, the very first artificial satellite, was launched by the Soviet Union in 1957, marking a significant milestone in space exploration. It served as a catalyst for advancements in satellite technology and paved the way for future space missions. In 2018, SpaceX made headlines by launching Elon Musk’s Tesla Roadster into an orbit around the sun, showcasing the capability of private companies to venture into space.
While Sputnik had a short-lived mission and eventually fell back to Earth a few months after its launch, Elon’s Tesla Roadster, now affectionately known as “Starman,” continues to journey through space as an iconic piece of celestial debris. It serves as a symbol of human ingenuity and exploration, reminding us of our presence beyond the confines of our planet.
Today, there are approximately 1,700 operational satellites orbiting Earth and beyond, serving various purposes such as communication, weather monitoring, navigation, and scientific research. These satellites form a vital part of our interconnected world, enabling global communication and providing invaluable data for scientific advancements.

But how exactly do we establish communication with these objects in space? Let’s take the example of a satellite placed in a geostationary orbit approximately 36,000 kilometers above Earth. When we want to send a command to such a satellite, the process involves converting the command into a radio wave. Radio waves are a type of electromagnetic radiation that travels at the astounding speed of 300,000 kilometers per second, matching the speed of light.
Once the command is transformed into a radio signal, a ground station equipped with a specialized antenna takes charge of transmitting the signal towards the satellite. This ground station antenna is responsible for beaming the signal with precision towards the target satellite.
Upon receiving the signal, the satellite’s sensitive antenna captures it, allowing the onboard systems to decipher and convert the signal into a code that the satellite’s onboard computers can comprehend. This code serves as instructions for the satellite to execute specific actions or transmit data back to Earth.
In most cases, satellites are equipped with both an uplink and a downlink, facilitating bidirectional communication between the satellite and Earth. The uplink is responsible for transmitting data or commands from the ground station to the satellite, while the downlink enables the satellite to send information or data back to Earth.

This intricate process of communication with satellites involves a combination of advanced technology, precise calculations, and highly skilled professionals who ensure seamless data transmission and command execution between Earth and space. As our reliance on satellites increases, so does the need for efficient and secure communication protocols, allowing us to further explore the depths of space and unravel the mysteries it holds.
Space communications for Apollo missions
These missions would not only venture farther into space than any previous endeavors but would also involve complex communication requirements. NASA would have to establish communication with three astronauts operating in two separate spacecraft, while simultaneously transmitting telemetry and live television images back to Earth.
To meet these challenges head-on, NASA developed a cutting-edge system known as the “Unified S-band.” This remarkable system was designed to handle various types of data, including telemetry, command signals, voice communication, and television transmissions. Each type of data was assigned its own frequency, but all of these signals were combined and transmitted through a single antenna. By consolidating the data streams, the Unified S-band system maximized efficiency and ensured streamlined communication between the Apollo spacecraft and the fixed ground stations strategically positioned across the globe.

With the implementation of the Unified S-band system, mission control gained the ability to maintain continuous contact with the astronauts throughout the duration of the mission. However, there was a brief interruption in communication when the spacecraft curved around the far side of the moon. During this period, commonly referred to as the “lunar blackout,” the signal between the spacecraft and Earth was lost until the spacecraft emerged on the other side approximately 40 minutes later.
To overcome this temporary communication loss, the astronauts had to execute a critical maneuver: firing up their engines. This crucial engine burn was necessary to ensure that the spacecraft would reappear on the expected trajectory on the opposite side of the moon. A successful burn would guarantee that Mission Control could precisely anticipate when they would regain communication with the Apollo 8 crew.
By meticulously coordinating the timing and execution of this engine burn, the astronauts ensured that their spacecraft would emerge from the lunar blackout and reestablish contact with Mission Control as planned. This meticulously orchestrated maneuver exemplified the precision and expertise required for successful communication and navigation during the Apollo missions, demonstrating the ingenuity and dedication of the entire NASA team.
The development of the Unified S-band system, along with the synchronized engine burn during the lunar blackout, exemplified NASA’s commitment to overcoming the unique challenges presented by the Apollo missions.
The Deep Space Network
Of course, this loss of signal can also happen to satellites orbiting Earth when the transmitter has no clear path to the satellite. To get around this, NASA set up the Space Network, which uses satellites in geostationary orbits to relay data from other satellites to fixed ground stations. This provides continuous global coverage for all Earth orbits.
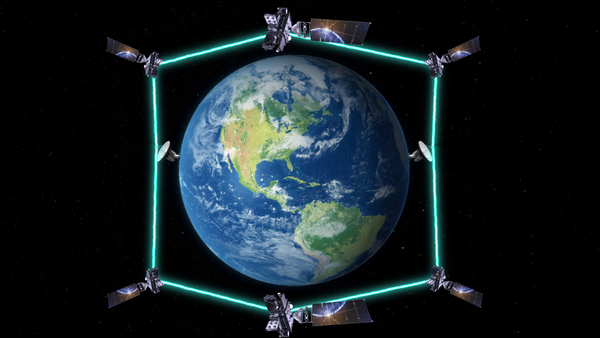
The Space Network, while encompassing satellites in Earth’s orbit, encounters limitations when it comes to communicating with satellites situated much farther away. When a satellite orbits Earth at a distance where the gravitational force exerted by the Sun equals that of the Earth, it reaches a maximum distance of approximately 1.5 million kilometers. However, what about satellites that extend billions of kilometers away from our planet?
The most remote man-made object from Earth is the Voyager 1 space probe, launched in 1977 with the primary mission of studying the outer regions of the solar system. Presently, Voyager 1 is an astonishing 20 billion kilometers away from Earth, hurtling through space at a staggering speed of 62,000 kilometers per hour. Given such vast distances, the transmitters employed by the Space Network lack the necessary power to effectively transmit and receive data from these deep space satellites. Consequently, an alternative solution called the Deep Space Network comes into play to enable communication with these distant satellites.
The Deep Space Network comprises antenna complexes strategically located at three different sites across the globe: in America, Spain, and Australia. These facilities are positioned approximately 120 degrees apart from one another on Earth, ensuring global coverage and enhancing the network’s capabilities. Each complex is equipped with a massive 70-meter antenna, capable of capturing and transmitting signals over immense distances, as well as two additional smaller high-efficiency antennas.

The utilization of such technologically advanced antenna systems in the Deep Space Network allows for improved communication with satellites situated billions of kilometers away. By harnessing the power of these sophisticated antennas, deep space missions can establish reliable and efficient links with Earth, facilitating the transmission of scientific data, images, and vital information that expands our understanding of the cosmos.
Satellites operating in proximity to Earth typically use low gain antennas, which spread their signals in every direction, making it relatively easy to pick them up here on Earth. These antennas are designed to provide wide coverage for efficient communication with ground stations. However, when it comes to satellites navigating the depths of space, a different approach is required. Satellites in deep space employ high gain antennas, which utilize focused beams to achieve a much stronger signal strength once it reaches Earth. These high gain antennas are carefully engineered to concentrate their radiowaves and enhance the signal reception capabilities over vast distances.
Deep space communication
In the case of deep space communication, achieving alignment between the antennas becomes crucial for successful data transmission. It involves the precise orientation and coordination of both the satellite’s high gain and low gain antennas, ensuring optimal signal acquisition and transmission. While the high gain antenna is responsible for transmitting and receiving data when precisely aligned with Earth, the presence of low gain antennas on deep space satellites serves as a backup mechanism. Even if the high gain antenna is not accurately pointing towards Earth, the low gain antennas provide a broader reception range, allowing ground stations to maintain contact and receive essential spacecraft telemetry.
The demands placed on deep space communication systems are continuously increasing as our exploration of the cosmos expands. A notable example is the Mars Reconnaissance Orbiter, which, as of March 2016, had already transmitted a staggering amount of over 300 terabits* of data back to Earth. To meet the escalating data transmission requirements, NASA anticipates that our space communications capability will need to grow by a factor of 10 in the next decade, as we launch more satellites into the vast reaches of deep space.

Therefore, as we continue to push the boundaries of space exploration, it is reassuring to know that these resilient spacecraft, sent to the farthest reaches of our solar system, possess the ability to establish communication links with Earth. Their ability to communicate back to us provides valuable insights into the outer realms of our universe, expanding our understanding and enabling further scientific discoveries.
Nice
nice
Your point of view caught my eye and was very interesting. Thanks. I have a question for you.