This is MIRI, the mid-infrared camera onboard the James Webb telescope. With this camera, Webb can see extremely distant stars and galaxies that are completely invisible to the human eye. This is only possible by cooling the camera down to just 6 degrees above absolute zero. But why does it need to be so cold?
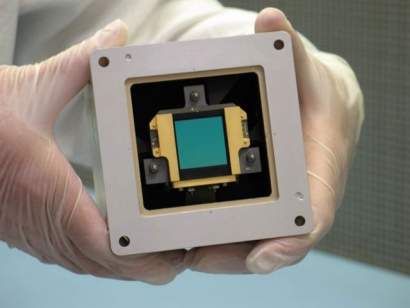
James Webb capturing infrared light
All of the cameras onboard James Webb detect infrared light, which is invisible to the human eye. As light travels through space, its wavelength is constantly being stretched. If something is far enough away, the light will be stretched so much that it is no longer visible by the time it reaches us.
This means there is a physical limit on how far we can see into space. Since MIRI’s sensors are made from arsenic and silicon, it can detect this super stretched infrared light and see beyond that limit.
These sensors work like regular camera sensors, by converting photons of light into an electrical signal. But in order to detect the faint signals of infrared light, the sensors on MIRI have to be extremely sensitive. Increasing the sensitivity however, introduces a lot of noise.
Whenever we point a camera at something, its sensor isn’t just detecting what we want it to see. There is so much more light bouncing around us that our eyes simply can’t detect. This can trick the pixels in the sensor into registering random levels of light, creating a layer of noise in the image. If the thing you are looking at has a bright enough signal, it will stand out much more compared to the noise.
However, if the thing you are trying to image is faint, like the infrared light from a galaxy, you’ll need to increase the sensitivity of the sensor, which in turn will drastically increase the noise. Since Webb is detecting infrared light, the problem gets even worse.
Every object in our universe emits heat energy, some of which is in the form of light. Most objects aren’t quite hot enough to emit visible light, but they do emit a lot of infrared light. The hotter the object, the more infrared light it will emit, which is essentially how thermal imaging cameras work.
Because of this, James Webb itself would emit so much infrared light that its sensors would be completely drowned out. And so, in order to limit the amount of infrared light produced by the telescope, its cameras need to be kept at a temperature of -234 degrees. But MIRI needs to be cooled all the way down to -267 degrees.
In order for MIRI to operate at -267 degrees, it’s located behind the massive 5 layer sunshield. This alone reflects so much heat and cools Webb’s cameras down to around -234 degrees. But since MIRI is much more sensitive than the other cameras, it faces an even bigger problem – dark current.
This is where the atoms inside the sensor itself vibrate and mistakenly register a photon of light, creating more noise. Since temperature is just a measure of how fast an atom vibrates, lowering the temperature will lower the vibration and therefore reduce the amount of dark current. Even at -234 degrees, the vibration of these atoms would be too much for MIRI’s sensor.
And so, they have to be cooled all the way down to -267 degrees, just 6 degrees above absolute zero. At this temperature the atoms in the sensor are almost completely still, drastically reducing the noise and allowing the faint infrared signals to shine through. But how did NASA take these sensors from -234 degrees all the way down to -267?
The typical method would be to carry a supply of compressed gas which could be released onto the detectors, cooling them down. This however takes up a lot of space and when the gas eventually ran out, the camera would become completely useless.
Instead, James Webb uses a closed loop system which constantly recirculates helium gas. Helium is used due to its low density, which allows it to expand and cool very quickly. The cooling process all starts here at the bottom of the telescope with a device called a pulse tube.
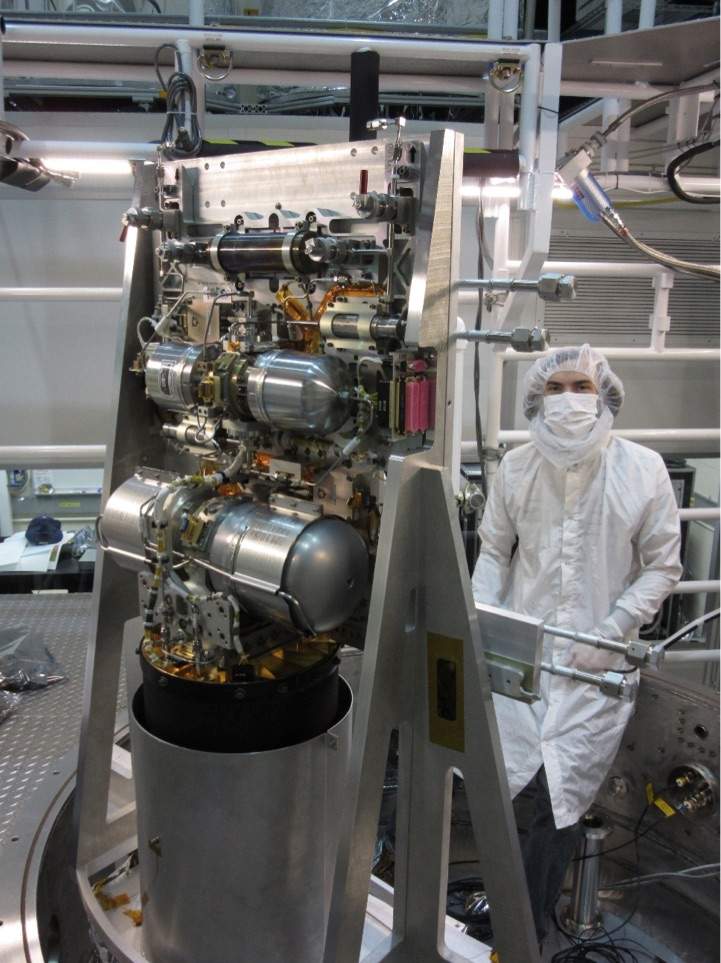
Inside these tubes are two pistons which move back and forth to compress the helium into the pulse tube. Since this movement would create a lot of unwanted vibration, these pistons have to move in the exact opposite direction with precision timing to cancel out each other’s movement. These pistons move very quickly just like a speaker to create a low frequency sound wave with a frequency of 30Hz.
This sound wave travels down the tube and gets compressed once it reaches the end, creating an area of higher pressure. Then, as it bounces back in the opposite direction, it expands, creating an area of low pressure before being compressed once again. If the next wave is sent out exactly as the previous one returns, it means the frequency perfectly matches that of the tube.
This creates a standing wave where the areas of high and low pressure remain in the same place. By tripling the frequency, it causes the waves to combine and create a stationary wave with multiple areas of high and low pressure. Since temperature and pressure are related, this also leads to areas of high and low temperature. This alone wouldn’t change the overall temperature because the hot and cold parts simply cancel each other out. But if there was a way to extract the hot parts, then the temperature would start to drop.
And so, 3 heat exchangers made of thin metal sheets are placed at the points where the hot and cold gas meet. These allow the gas to pass through whilst also absorbing some of its temperature, causing a heat gradient to form. The heat from the hot side is pulled out and sent to the radiators via a heat exchanger.
Then, the cold temperatures on the other side are also drawn out. This causes the temperature to drop from 27 degrees all the way down to -256 degrees at the final heat exchanger. But how is this used to cool the sensors located at the opposite end of the telescope?
Next to the pulse tube is another set of pistons that compress helium in a completely separate line of tubing. This line passes through the cold parts of the pulse tube’s heat exchangers, cooling the helium all the way down to around -256 degrees. But still the helium isn’t quite cold enough.
From there, the helium goes on a long journey, winding its way up through 10 meters of thin tubing until it reaches the cold head assembly. Inside there is another heat exchanger and a tiny hole less than 1 millimeter in diameter. The helium is pushed through the hole and undergoes something called the Joule Thomson effect. As the gas moves through the hole it gets compressed before quickly expanding on the other side.
This rapid expansion causes the pressure to drop, cooling the gas very quickly. This is why blowing air through our mouth is colder if we make a smaller hole with our lips. On James Webb, this cools the helium all the way down to just -267 degrees. From here, the helium flows onto copper plates attached to the back of MIRI’s sensors, cooling them down to the required temperature.
After that the helium has done its job and it flows back down the telescope where the entire process begins again. When NASA began designing James Webb, no cryocooler with this level of cooling existed, and so engineers had to really push the boundaries of physics, all whilst conforming to the limits of fitting it into a space telescope.
Great video! Unfortunately to the best of my knowledge the explanation of the Joule Thompson effect isn’t quite accurate in the final cooling stage, though I could be wrong. Blowing air through your mouth is not related to the Joule Thompson effect as it only applies to real gases at extremely low temperatures (such as this case) or very high pressures. Additionally, it’s not due to the rapid lowering of pressure that it cools but rather that the rate of which the pressure decreases is lower than that of which it’s volume increases. In the Joule Thompson equation H = U + PV, the enthalpy is constant, thus the total internal energy (hence temperature) would only decrease if PV increased, and the only way for PV to increase given a reducing pressure is for the volume to increase at a higher rate (which occurs as a result of its intermolecular forces).